Cracking the Code: How Genetic Sequencing Uncovers Hidden Mutations in Your DNA
From understanding hereditary diseases to predicting drug responses, sequencing helps researchers uncover the subtle language of life
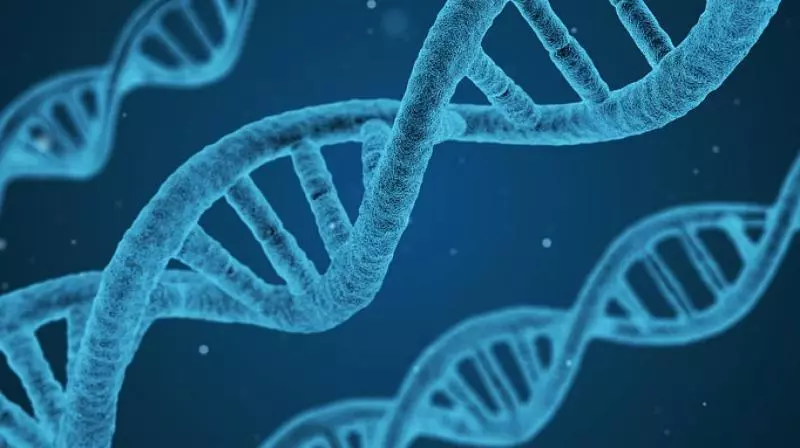
Genes in the millions of cells throughout the human body aid in the construction, repair, and maintenance of all bodily functions. However, mutations in these genes can disrupt this balance, leading to various diseases or affecting how our bodies adapt to diverse environments. However, it is important to note that genetic variations are not always harmful, they also help us understand how genes function in our bodies and influence traits such as height, metabolism, immunity, and more.
To find such hidden stories of our DNA, scientists and researchers rely on ‘sequencing techniques’ – powerful tools decoding genetic information and biological data unique to each individual. From understanding hereditary diseases to predicting drug responses, sequencing helps researchers uncover the subtle language of life. As we celebrate National Science Day this year, let’s explore how various sequencing techniques are revolutionizing science and healthcare, by enabling us to uncover more sequenced variants and gain deeper insights into how genes function.
- Whole Genome Sequencing (WGS): As the name suggests, WGS is a comprehensive approach to identifying and studying an organism's entire DNA sequence. This information is critical for diagnosing genetic disorders, investigating mutations linked to cancer development, and monitoring disease outbreaks. Advancements in WGS have been driven by large-scale global genomic initiatives, such as GenomeAsia 100K, GenomeIndia, 1000 Genomes Project, UK Biobank, All of Us, and TOPMed. These projects are providing deeper insights into human genetic diversity, facilitating rare disease identification, and advancing precision medicine. By converting each gene into digital data, WGS enables personalized therapy, population genetics research, and improved public health strategies, bringing us closer to a future where medicine is truly tailored to an individual’s genetic makeup.
- Sanger Sequencing: Named after Nobel laureate Frederick Sanger, this technique was the original pioneer in DNA sequencing. Sanger sequencing decodes genetic information one strand at a time, providing high accuracy for smaller DNA fragments. While it is less commonly used today in large-scale studies, it remains the gold standard for validating other sequencing results. Sanger sequencing is still widely used in clinical diagnostics to confirm the presence of specific mutations, especially in conditions like cystic fibrosis and hereditary cancers. Its precision and reliability continue to make it a cornerstone of genetic research.
- Next-Generation Sequencing (NGS): NGS technology, a breakthrough in precision medicine, can test multiple samples and multiple targets simultaneously, enabling the investigation of numerous mutations in a tumor sample quickly and cost-effectively. In recent years, next-generation sequencing (NGS) has begun to replace Sanger sequencing, primarily because it can sequence millions of DNA fragments simultaneously in contrast to Sanger’s ability to only analyse short pieces. NGS has become a game-changer in identifying variants in complex diseases and lifestyles diseases, such as diabetes and cardiovascular conditions. It is also widely used in oncology for tumor profiling, enabling targeted therapies that can significantly improve patient outcomes.
- Single-Cell Sequencing: Single-cell sequencing enables researchers to examine the DNA or RNA of individual cells, rather than averaging genetic information from a larger sample. Even within the same tissue, individual cells can exhibit significantly variation in their genetic activity and functions. This method isolates single cells and amplifies their genetic material to investigate the variations between them. It is important for studying cell development, identifying rare cell populations, and examining tumor heterogeneity in cancer research.
- Long-Read Sequencing: Long-read sequencing provides a more detailed view of complicated regions, such as structural variations or repetitive sequences. This approach is particularly useful for studying rare genetic diseases, as conventional methods may to identify the underlying cause. One of the biggest breakthroughs enabled by long-read sequencing is the assembly of new genomes with unprecedented accuracy. It has been instrumental in generating gapless, complete human genome sequences, such as the Telomere-to-Telomere (T2T) Consortium’s achievement of assembling the first fully sequenced human genome. Additionally, long-read sequencing has also proven useful in agriculture, aiding in the study of plant genomes to improve crop resilience and productivity.
- Methylation Sequencing: Genetic code sequencing reveals "what's written," whereas methylation sequencing explains "how it's read." Methylation is an epigenetic modification that alters gene expression without changing the DNA sequence itself. This method is crucial for studying disorders like cancer, where abnormal methylation patterns can silence tumor suppressor genes or activate oncogenes. Methylation research has also provided valuable insights into aging, development, and environmental factors that influence our DNA, resulting to a more comprehensive understanding of health and disease. One of the most groundbreaking applications of methylation sequencing is in early cancer detection. Many healthcare companies are leveraging this technology to develop liquid biopsy-based screening tests capable of detecting multiple cancers from a simple blood sample, even before symptoms appear.
Expanding Beyond Genomics: The Role of Multiomics
Multiomics is essential for understanding how genetic factors contribute to the shift from health to disease. While sequencing alone can identify genetic variants, many alterations require a more comprehensive approach. Integrative genomics encompasses methods like RNA sequencing, protein analysis, and the study of biochemical pathways. In cancer research, sequencing can identify mutations in oncogenes, whereas multiomics provides a broader understanding of how the expression of guidance factors, transcription factors, and signal transduction components changes in response to tumor growth. Similarly, in neurodegenerative diseases like Alzheimer’s, multi-omics can help link genetic risk factors with changes in brain chemistry, aiding early detection and improving therapeutic strategies.
As technology advances, researchers are working to analyze biological systems in more informative ways. The combination of sequencing technologies and multiomics approaches helps achieve this goal. These systems provide more detailed information, leading to accurate diagnosis, enhanced drug discovery, and personalized treatment strategies.
By Dr Ravi Gupta, Vice President - Bioinformatics, MedGenome